Mechanosynthesis

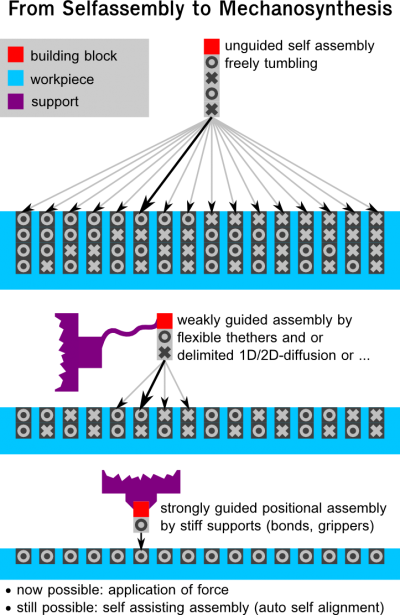

Mechanosynthesis is a method of assembly. It is the process of assembling atomically precise components to larger atomically precise structures by pick and place methods. For information about almost atom-by-atom synthesis involving large applied forces see: piezochemical mechanosynthesis instead.
In Nanosystems a definition with very loose requirements is given:
Mechanosynthesis is "molecular synthesis directed by mechanical means"
And it was really meant this general way as can be seen on the page "Pathway controversy".
There is a bit of a problem with a community consensus on the meaning of the term "mechanosynthesis".
For details see: Mechanosynthesis (disambiguation)
Anyway. On this wiki "mechanosynthesis" ...
- ... refers to anything that involves positional assembly.
- ... may or may not feature the capability of applying high local forces (piezochemistry).
- ... will be avoided in favor of more specific terms.
- In particular the far term target will be referred to with "piezochemical mechanosynthesis".
- When thermally driven self assembly aspects are still present (beside the necessary positional assembly) then "semi-mechanosynthetic" or better "semi-positional" may be used.
Kinds of mechanosynthesis
Piezochemical mechanosynthesis (the far term target)
See main article:
- Piezochemical mechanosynthesis – Also: "(High) force applying mechanosynthesis"
- And in particular Piezochemical mechanosynthesis#Surprising Facts
Examples:
- Synthetic: Mechanosynthesis of diamond with stiff manipulators
- Natural: Enzymes that use chemical energy (e.g. ATP) on one site transport the energy mechanically through the protein and add a part to a workpiece on the encymatic site (Ligases, ATPases, ...)
Site activating semi-mechanosynthesis
Mechanical force is used only to make an otherwise unspecific site ractive. The actual building blocks are washed in after activation and attach to the activated sites completely unguided. Then the cycle repeats.
Strongly directed mechanosynthesis (strongly guided AP-block assembly)
There is fully non-specific binding between components.
That is: Components do not encode information about their target position (target position in the target structure) in their shape.
Guided assembly of atomically precise blocks is ...
- ... more or less equivalent to positional assembly without additional capabilities.
- ... a form of mechanosynthesis according to how the term "mechanosynthesis" is interpreted on this wiki.
- when interpreting mechanosynthesis as equivalent to piezochemical mechanosynthesis (not done in this wiki) then this is not mechanosynthesis.
Self assisting assembly (that is thermally driven final self alignment) can still be used.
Examples for guided assembly of atomically precise blocks:
- Synthetic: pick and place DNA bricks or assemblies to specific locations (not done yet)
- Synthetic: pick and place diamondoid molecular elements to specific locations
Side note:
In this kind of assembly there also can be quite considerable forces involved (VdW forces).
But those are pretty low on a per-bond-level and do not change the nature of the molecules.
That is usually no covalent bonds are formed or broken. (There may be exceptions.)
Supra-molecular mechanosynthesis (weakly guided AP-block assembly)
Weakly guided assembly that relies on weak bonds.
Since it's not strongly guided (e.g. block on a tether) directed force fundamentally can't be applied.
Like it's fundamentally impossible to push on a string/tether (excluding inertial throwing which does not work at the nanoscale)
It is questionable whether this should be be counted to the methods of mechanosynthesis. this form of assembly forms a continuum to true self assembly. Examples:
- Natural: Carrying a protein on a flexible polymeric tether to a location for local selfassembly
- Synthetic: Carrying a structural DNA brick on a tether to a location for local self assembly (not done yet)
Related: Modular molecular composite nanosystems (MMCNs)
The piezochemical mechanosynthesis threshold
Starting with piezochemical mechanosynthesis right away proved to be very challenging to scale. (See: Direct path). Easier might be to start with self assembly, move on to weaker types mechanosynthesis and continuously improve on that. (See: Incremental path).
When moving from more limited capabilities of atomically precise placement of larger blocks (where only rough placement is needed and the rest is snap in place by complementary surfaces and intermolecular forces) towards more advances capabilities there comes a point where one one "suddenly" can reliably target single atoms. At this point one can start to apply forces to directly influence chemistry in a very controlled fashion (piezochemical mechanosynthesis).
(wiki-TODO: add illustrative image)
Supercritical lattice scaled stiffness
The suitability of an atomic material (or pre-assembled block metamaterial) to place units of itself via manipulators made from itself can be quantified by the quantity of "lattice scaled stiffness". That is the relation between the stiffness of the material and the spacing between its snap-in-points. Given an intended operation temperature one can judge feasibility and reliability for the material. In quantitative terms: "error rates".
Only if error rates are low enough the material is suitable for mechanosynthesis. One could say the material has "supercritical lattice scaled stiffness".
(wiki-TODO: add illustrative image)
Mechanochemical parameters
- t_act … actuation time (toward and away from reactive geometry) – (piezochemical: ~10^-6s => ~1MHz)
- t_trans … transformation time (geometry permits reaction to occur) – (piezochemical: ~10^-7s)
- k_react … reaction rate (probability for reaction per time during t_trans wile not yet occurred)
- k_isc … inter system crossing rate
- => P_err … likelyhood of error per reaction – (piezochemical: smaller than 10^-15)
Source: Nanosystems 8.3.1.b Mechanochemical parameters (P197)
Details
Basic mechanosynthesis
Brownian mechanosynthesis
A mild form of mechanosynthesis can actually be done without active drive mechanisms and without full robotic control.
In fluid/gas phase a reaction between two reagents occurs only when a correct three dimensional position plus a correct three angle orientation is reached at the same time "by accident" through thermal vibrations (self assembly). When one hooks the reagents to a reasonably stiff common hinge or rail and letting the thermal motion drive the "mechanism" only one correct angle or one correct position must be reached "by accident" which is much more likely and will thus happen much more frequently. This way one can make the rate of encounter rise for wanted and diminish for unwanted reactions. In more general terms: Through confinement of reagents into a sub volume (partial machine phase) the effective concentration of the reagents increase and the reaction rates rise.
More information on brownian mechanosynthesis and and effective concentration on Eric K. Drexlers Blog:
- Motors, Brownian Motors, and Brownian Mechanosynthesis (archive); (old dead link);
- Effective Concentration in Self Assembly, Catalysis, and Mechanosynthesis (1) (archive); (old dead link);
- Effective Concentration in Self Assembly, Catalysis, and Mechanosynthesis (2) (archive); (old dead link)
On the way from brownian transport and self assembly to the most weak forms of mechanosynthesis three things can be identified that can be used to characterize the method. This would be:
- dimensionality: 3D (in full space) / 2D (on a bent sheet) / 1D (on a twisted rail) / due to lots of folds and curvature in between in a quasi fractal dimension
- constrainedness: e.g. -- arbitrarily formed tubes or bubbles in 3D -- arbitrarily shaped stripes or patches in 2D -- (constraints can not only be provided by walls but also by tethers)
- directionality: some degree of suppression of backward hops on every step. (wiki-TODO: add links to: arrow of time, efficiency, thermodynamics, ...)
(full control) mechanosynthesis
Fully controlled mechanosynthesis normally simply called mechanosynthesis controlls all degrees of freednom allowing only for thermal vibration amplitudes determined by the stiffness of the controlling structure. Demonstration experiments with scanning probe microscopy would be useful for every technology level from I to III. Mechanosynthesis has been demonstrated for non metals at room temperature already. In most cases the used tooltips where not atomically precise and if not reversibly rechargable.
Of basic mechanosynthesis with molecular blocks as done in technology level I not much is known yet.
[Todo: investigate what experiments have been done - experimental examples needed!]
Experiments that could be done:
- Create big stiff AP molecular building blocks by self assembly (e.g. stuctural-DNA-Bricks) such that they expose complementary surfaces
- Try to put them together and measure the strength of the formed bond
Mechanosynthesis in technology level II needs to use templating cores.
Advanced mechanosynthesis
In advanced forms of mechanosynthesis technology level III components get assembled to structures utilisizing tooltip chemistry. It's feasibility was demonstrated theoretically and experimentally for nonmetals at room temperature. It shall be performed in the many robotic mechanosynthesis cores of all advanced APM systems.
Mechanosynthesis of diamondoid molecular elements
Putting things together directly from the ultimate construction toy.
Unstrained DMEs (out of e.g. hydrocarbon or hydrosilicon) are the structures easiest to produce by mechanosynthesis. Since only a few (still quite a lot) tooltip chemistry steps need to be understood and implemented. Unstraind structures though cannot approximate cylindrical structures well and thus form poor bearings. Exclusive hydrogen passivation also leads to higher friction between sliding surfaces. See: "superlubrication".
Strained structures like bearings need additional pre-produced tools. E.g. a ring shaped part like a bearing could be produced in two halves. In general either those two parts go less or more than 360° around. If less the two halves can be merged at one side and pressed together by a prepaired tool to merge at approximately the other side. If a little more than 360° the two halves might be simply pressed together by the special tool. If much more an additional widening tool is needed.
An alternate method for the creation of strained shell structures may be to start with an unstrained but curved single atom with ring on a template surface (possibly a pre-built strained shell structure) extend it radially then vertically and finally break / pluck it off the template.
Moving parts need to be temporarily tacked down by some means while in the building phase.
Mechanosynthesis of less stiff AP structures
Mechanosynthesis of less stiff but still highly standardized structures should be possible by temporarily restraining the products degrees of freedom at the building suite. Long nanotubes could be fed through a pre-produced hole, bigger sheets of graphene through a slit.
Built order must be chosen such that "overhangs" never form thin floppy walls. This may require placement mechanisms with more than three degrees of freedom (which are likely to be required for e.g. pi-bond breaking processes anyway).
In some cases a floppy chain can be built by keeping it tensioned and only extending it near the tip. [Todo: note reaction sequence that shows this]
Mechanosynthesis of special structures
In most situations atoms don't behave like building bricks of specific shape. It's just that when doing mechanosynthesis one carefully choses the ones that do and choses the configurations in which they do. Thus its no wonder that one occasionally runs into situations where things get complex. An example is nitrogen when it's used as dopant atoms in diamond. One bond is missing to four. When depositing it to the growing surface you might have to choose an orientation [to verify]. When it's finally fully and symmetrically embedded in diamond its orientation will be lost. Another example are boron and aluminum. They can form electron deficiency bonds that can behave in unexpected ways.
Then there's hybridisation of elements of some elements from the second period namely carbon nitrogen and oxygen. Prime example are the components for the "rod logic" (nanomechanical computation) presented in Nanosystems (polyyne control cables with attached knobs). Not only do they have limited stiffness (see above) but also carbon in different hybridization states. (TODO: check research status on how far hybridisation can be controlled)
Several metal oxides and other new diamondoid materials will be of interest but pose significant effort for the design of the whole capture preparation placement chain.
For more the main article on: "Limits of construction kit analogy".
Further notes
Somewhat complementary to mechanosynthesis is "atomically precise disassembly". Note that this is much harder task. Mechanosynthesis is not reversible by simply reversing the motion trajectories. If even the location of the atoms in the chunk of material to disassemble are unknown this problem becomes incredibly difficult. Luckily "atomically precise disassembly" is not needed for fully fledged diamondoid APM system. On the downside this could lead to recycling problems.
Complementary to the capture of resource molecules is the creation of small oxide and hydride moieties when diamondoid waste is burnt (controlled oxidation) or cracked (hydration). See: "hot gas phase recycling cycle"
(TODO: Mechanosynthesis can be made to be highly energetically reversible (efficient). How does this relate to reversing the reaction?)
Start of an answer: Low speed efficiency limit
Development of increasingly advanced artificial mechanosynthetic capabilities
- (wiki-TODO: add infographic showing stages with increasing lattice scaled stiffness. from foldamers over large lattice biominerals to diamond & co)
- (wiki-TODO: more on effective concentration)
Related
- Spectrum of means of assembly
- Piezochemical mechanosynthesis or force applying mechanosynthesis (possible shorthand forcosynthesis)
- Experimental demonstrations of single atom manipulation
- Mechanosynthesis core
- Tooltip chemistry in machine phase aka machine phase chemistry; (robotic "stereotactic" manipulation – positional assembly)
- What it takes for advanced mechanosynthesis to provide practical levels of throughput: Atom placement frequency
- The two infamous points of critique: Fat finger problem & Sticky finger problem
But there are two more. See: The finger problems - The harder problem of inverse mechanosynthesis: Atomically precise disassembly
- Positional assembly as the here present Method of assembly
- A path towards advanced mechanosynthesis: Introduction of total positional control
- Atomically precise nanoscale assembly without mechanosynthesis: Thermally driven assembly & Diffusion transport
- Raising efficiency of advanced mechanosynthesis: Dissipation sharing & Low speed efficiency limit
- weaker Topological atomic precision vs stronger Positional atomic precision
- An important aspect of advanced mechanosynthesis: High pressure
- Lattice scaled stiffness & Effective concentration
External links
- Ralph Merkle and Robert Freitas, in a joint session discuss diamond mechanosynthesis at the 2007 Foresight Vision Weekend Unconference.
video | text (dead link - replacement needed) | text (via waybackmachine) - A Minimal Toolset for Positional Diamond Mechanosynthesis
from Robert A. Freitas Jr. and Ralph C. Merkle - Institute for Molecular Manufacturing, Palo Alto, CA 94301, USA - About advanced mechanosynthesis: Annotated Bibliography on Diamond Mechanosynthesis
- Demonstration of vertical manipulation of single Si Atoms at ~70K: [1]
- There's no problem of "fat fingers" or "sticky fingers" [2]
- Eric Drexlers Blog: From Self-Assembly to Mechanosynthesis
- example of supra-molecular mechanosynthesis:
single molecular layer of PTCDA (leave to Wikipedia - please come back again) on Ag(111)
Patterning a hydrogen-bonded molecular monolayer with a hand-controlled scanning probe microscope
- Wikipedia: Mechanosynthesis
- Wikipedia: effective concentration (aka thermodynamic activity)
Table of Contents
Contents
- 1 Kinds of mechanosynthesis
- 1.1 Piezochemical mechanosynthesis (the far term target)
- 1.2 Site activating semi-mechanosynthesis
- 1.3 Strongly directed mechanosynthesis (strongly guided AP-block assembly)
- 1.4 Supra-molecular mechanosynthesis (weakly guided AP-block assembly)
- 1.5 The piezochemical mechanosynthesis threshold
- 1.6 Supercritical lattice scaled stiffness
- 2 Mechanochemical parameters
- 3 Details