Difference between revisions of "Colonization of the solar system"
(→From innermost outwards: Added info to Galileian moons) |
(→External links: added links to wikipedia pages about the moons of the gas giants) |
||
Line 160: | Line 160: | ||
* [https://en.wikipedia.org/wiki/List_of_trans-Neptunian_objects List of trans-Neptunian objects] | * [https://en.wikipedia.org/wiki/List_of_trans-Neptunian_objects List of trans-Neptunian objects] | ||
---- | ---- | ||
+ | * [https://en.wikipedia.org/wiki/Galilean_moons Galilean moons] and the other [https://en.wikipedia.org/wiki/Moons_of_Jupiter Moons_of_Jupiter] | ||
+ | * [https://en.wikipedia.org/wiki/Moons_of_Saturn Moons of Saturn] | ||
* [https://en.wikipedia.org/wiki/https://en.wikipedia.org/wiki/Moons_of_Uranus Moons of Uranus] | * [https://en.wikipedia.org/wiki/https://en.wikipedia.org/wiki/Moons_of_Uranus Moons of Uranus] | ||
+ | * [https://en.wikipedia.org/wiki/https://en.wikipedia.org/wiki/Moons_of_Neptune Moons of Neptune] |
Revision as of 21:32, 30 June 2021


Up: Spaceflight with gem-gum-tec
This page is about how advanced atomically precise manufacturing may be usable to colonize interplanetary space. The focus is on possibilities that are relatively near term (under a century). If more far term stuff comes up this will be especially noted.
Contents
List of objects in our Solar system
Bodies with dense atmospheres are marked with a star: ★
Atmospheres can deliver building materials in the easiest processable form.
Unlike mining in rock:
- Elements come in form of molecules that all have exactly the same "standartized" shape.
- The material comes to you rather than you needing to come to the material.
Plus atmospheres give radiation protection and allow for aerial transport.
For more about using atmospheres as a resource
- See: Air using micro ships (and macro ones).
- See: Air as a resource (specific to Earth)
From innermost outwards
- Mercury (planet) (the tiniest of our planets) - hard to reach because high delta-v from earth
- ★ Venus (12,103.6km) – is perhaps a paradise for advanced gemstone metamaterial technology.
- ★ Earth (12,736.5km) – Related: Seasteading
- ★ Mars (6,792.4km) – Thus mars is the second tiniest "normal" planet right after Mercury.
Mars has an rather thin atmosphere (6.28mbar) that for human physiology equates to a full vacuum (pressurized spacesuit unconditionally needed).
But it is still plenty thick enough to be usable as a building material for gemstone metamaterial technology.
Asteroid belt – advantages: big surface and area low gravity
- Ceres (~940km) - nearest "waterworld" to Earth
- Psyche (278±5 × 232±6 × 164±4)km – the biggest metallic planetary core remnant
Gas giants – advantage: atmosphere – disadvantage: enormous gravity and high radiation
- ★ Jupiter, Saturn, Uranus, Neptune. See: Gas giants
Jupiter:
- Io (~3660km) – extreme volcanic activity (mainly hot molten sulfur) and extremely radiation grilled – only the deep depths of the gas giants seem more unsurvivable
- Europa (~3120km) – cryovolcamic activity – Skyscraper high ice spikes (penitentes) supspected – very strongly radiation grilled
- Ganymede (~5270km) – still quite high radiation but much less than Europa
- Callisto (~4820km) – that one might actually be a nice place for early colonization. It's the most geologically dead one of the four though due to the least tidal hating.
Saturn:
- ★ Titan (~5150km) – is Saturns only giant moon and the only Moon in the solar system with a notable atmosphere. It surpasses the one of Earth in terms of both density and pressure. Atmosphere blocks space radiation.
Uranus:
- Aliel & Umbriel – both around ~1150km
- Titania & Oberon – both around ~1550km
Neptune:
- The only giant moon there is Triton (~2700km) – cryovolcanism
- Transneptunian objects like Pluto and Charon
Smaller spherical moons
All smaller but still mostly spherical moons (diameters heavily rounded):
- Jupiter: – Biggest smaller moon right after the for giant moons is Amalthea ~170km (250 × 146 × 128) – it is already far from spherical
- Saturn #1: – Minas ~400km, Enceladus (~500km), Thethys (~1060km), Dione (~1120km), Rhea (~1530km), Titan (~5150km), Iapetus (~1470km)
- Saturn #2: – Hyperion ~270km (360 × 266 × 205) – first moon that id quite far from round – all other ~200km and below
- Uranus: – Miranda (~470km), Ariel (~1160km), Umbriel (~1170km), Titania (1580km), and Oberon (~1520km)
- Neptune: – Proteus ~420km (436 × 416 × 402), Triton (~2700km), Nereid (~360km) – all other ~200km and below
Interestingly there are no objects in our solar system with liquid nitrogen oceans on the surface. Titan escapes that fate barely.
Also there are no ones with and liquid hydrogen/helium on the surface (this would need to be big objects very far out so it may be less certain).
Asteroids in the main belt between Mars and Jupiter
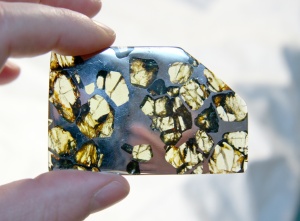
- pro: no gravity traps
- pro: enormous accessible surface area - probably way greater than all the planets and moons together
- pro: just the right temperature for the presence of a variety of materials => …
- pro: … most unobstructed access to the perhaps the most interesting and useful group of elements
lithophile element, chalkophile elements, and a maybe somewhat limited amount of atmophile (aka volatile) elements.
Meteoroids coming from these (and recent space missions 2020) give us info about the element distribution to expect.
See: Carbonaceous chondrites
- con: all the material is in the solid state requiring complex mining.
Well Ceres seems to have some subsurface briny water inside. - con: there is quite a bit less solar energy than on earth - but it is still enough to be useful
- con: laggy telecommunication in a dispersed net due to light-speed run-times
M-type asteroids
These asteroids are essentially pieces of smashed up/open proto-planetrary cores.
They are giving us the only option to physically access pretty much the same material environment that is present very very deep within out earth.
A material environment that will likely remain inaccessible even with advanced APM available. Check out the deep drilling page for details).
Setting up base at one of these asteroids would/will allow access to huge amounts siderophile elements (see Goldschmidt classification) These elements include Gold Au Platinum Pt and Iridium (among others) and are much more scarce on earth because they are highly depleted in our Earths crust.
Still, most interesting elements for large scale construction with advanced APM remain the most common ones.
Iron: First and foremost in M-type asteroids there is are huge amounts of iron.
This is presumably due to iron having the lowest energy per nucleon and thus being the "nuclear ash" final end product that cannot be further fusioned or fissioned any further.
(wiki-TODO: maybe add the classic energy per nucleon chart for illustration here)
And of course due to its high specific weight making it sink to protoplanetrary cores (aka the process of "differentiation").
- Con: Iron does not form very strong gemstones with oxygen.
- Pro: In space iron does not rust since there is no oxygen around.
- Pro: Also as a metal with an electron gas iron may feature a bit more self healing after mild radiation hits.
- Con: Pure metals such as iron are not optimal for APM but they can be used as long as some conditions are met which are:
- -- (1) for preventing surface diffusion: operation at low temperatures (e.g. far out the solar system) and/or flat surfaces
- -- (2) to prevent irreversible seamless welding: keeping nanoscale surfaces from coming too close together
Nickel: Nickel is usually mentioned as the second most common element in earths core but …
When checking out the compositions of metallic meteroids it seems that there is actually very little nickel in there and athere elements take second place.
(TODO: investigate what is going on here a bit deeper)
(TODO: list most common elements beyond iron)
Bodies in the outer solar system
Here considered outer solar system: Jupiter beyond [[1]] all the way out to the Oorth cloud.
These are usually covered in thick sheets of structurally mostly useless ices like water ice ammonia ice or (when really far out) even methane ice and nitrogen ice. One exception to this "rule" is Jupiters innermost giant moon Io which got/gets tidally heated so much that it volcanically evaporated off all of its volatile elements despite its large distance to the sun. At least that is the naive conclusion from observations.
The only in bulk structural useful ices from volatile elements are dry-ice (CO2) an methane-ice (CH4) due to their carbon content. Titans Hydrocarbon lakes can be counted to that.
Moons and dwarf planets in the outer solar system
Furter out in the solar system small bodies become increasingly icy. Water ice and at some point even nitrogen ice becomes rock forming material. If not enough carbon and silicon is present one might want to mechanosynthesize weaker bonding ices there and use those materials for not too demanding structural purposes
Flavors of diamondoid gem gum technology
Vastly differing chemical and thermal conditions at different places in the solar system could lead to differentiation (do not use "speciation"!) of diamondoid technology into very different branches.
Structures built out of water ice via cryonic inter-molecular mechanosynthesis wont find much use beside ephemeral consumables on earth since they quickly melt when uncooled or diffuse when insufficiently cooled. Further out and farther from the sun though ice and other compounds that are volatiles on earth can be seriously used as permanent building materials. This materials are also the most abundant materials in those regions so they are likely to be used.
Unlike methane water can't be safely polymerized to stuff that does not melt above 0°C. Long peroxide chains are a powerful explosives. Also oxygen polymers are un-branched linear chains and thus can't form tight meshed poly-cyclically looped covalent stiff diamondoid materials. So technology that uses only the elements oxygen and hydrogen for structural components (that is water) stays out there. Reasonably safely making explosive crystals from mostly water that do not melt will certainly be possible via mechanosynthesis since they can be made practically perfectly clean - the usefulness may be questionable.
- Chemically reducing environments (nonoxidic compounds)
- high temperature environments (refractory materials)
- metal rich environments (planetary core material in the asteroid belt)
Related
- gem gum spacesuits a special class of gem gum suits
- Telepresence robots based on multi limbed sensory equipped shells
External links
Wikipedia:
- Space colonization
- List of natural satellites
- List of exceptional asteroids
- List of trans-Neptunian objects