Difference between revisions of "Chemomechanical converter"
(moved entropic batteries section to entropomechanical converters section) |
|||
Line 1: | Line 1: | ||
− | A nanomechanical mechanism that converts chemical into mechanical energy and vice versa. <br> | + | A nanomechanical mechanism that converts chemical into mechanical energy by splitting or joining covalent bonds and vice versa. <br> |
− | Subclass of [[molecular power mill]]s. | + | Subclass of [[molecular power mill]]s. [[Entropomechanical converters]] like entropic batteries use similar zip style reactor cells. |
Chemomechanical motors of [[technology level III]] always come with their storage. | Chemomechanical motors of [[technology level III]] always come with their storage. | ||
Line 39: | Line 39: | ||
Note that like in a hydrogen system a complete radical battery systems include both an [[energy storage cells|energy storage]] and an energy converter (reactor). The generated driving motion can either be delivered to electrostatic motors / generators or in one or more steps [[macroscopification|merged]] to an axle of macroscopic scale. Its desirable to pack the functionally into different parts (e.g. different [[microcomponents]]). This allows adjustable storage to converter ratios. | Note that like in a hydrogen system a complete radical battery systems include both an [[energy storage cells|energy storage]] and an energy converter (reactor). The generated driving motion can either be delivered to electrostatic motors / generators or in one or more steps [[macroscopification|merged]] to an axle of macroscopic scale. Its desirable to pack the functionally into different parts (e.g. different [[microcomponents]]). This allows adjustable storage to converter ratios. | ||
− | |||
− | |||
− | |||
− | |||
− | |||
− | |||
− | |||
− | |||
− | |||
− | |||
− | |||
− | |||
− | |||
− | |||
− | |||
− | |||
− | |||
− | |||
− | |||
− | |||
− | |||
− | |||
− | |||
− | |||
− | |||
− | |||
− | |||
− | |||
− | |||
− | |||
= Controlled molecular recombination = | = Controlled molecular recombination = |
Revision as of 13:38, 3 May 2014
A nanomechanical mechanism that converts chemical into mechanical energy by splitting or joining covalent bonds and vice versa.
Subclass of molecular power mills. Entropomechanical converters like entropic batteries use similar zip style reactor cells.
Chemomechanical motors of technology level III always come with their storage. Since the electrical intermediate step of todays systems is avoided "batteries" and motors overlap here. Since they also avoid the thermal intermediate of todays combustion engines they have no fundamental limit on efficiency. Storage and reactors can potentially be combined in any ratios. See energy storage cells.
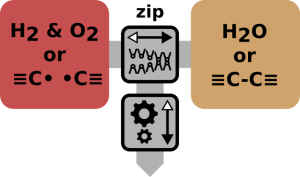
Radical Batteries
They consist of stored nano sized gas-tight sealed cells and a reactor. In the cells carbon (or silicon) atoms have each three bonds to one corresponding handle and a single bond to an opposing equal but mirrored part. The single bonds act as reversible predetermined breaking points. Some nano-mechanical mechanism outside the sealed cells (the reactor or chemo-mechanical converter) is able to grasp the handles of an incoming cell pull them apart and put them into a lock position. The result is an energy loaded cell with some pairs of high energetic C-radicals (C atoms with open broken bonds) inside. The breaking process evens out the nonlinearity of the single bonds by breaking / building many bonds at the same in a zip style fashion and also handling multiple cell at the same time too. The breaking process furthermore transforms the motion into a wider ranging movement with less force (lever effect / gearing up). This way single bonds are not jerkily ruptured which would waste energy as heat.
unbonded #### bonded Chained \|/ #### ########### C \|/ \|/ \|/ \|/ • C C C C | | | | • C C C C C /|\ /|\ /|\ /|\ /|\ #### ########### ####
Unlike thermal engines with a radical-zip-battery one can directly convert from chemical to mechanical energy without the detour over thermal energy. Without this detour (which would immediately split up the yielded energy into recoverable free and irrecoverable bound energy) we are no longer bound by the maximum efficiency of an carnough-process and can get (within limits) arbitrarily near 100% reversibility. The key to this is the complete positional control over every reactant (aka machine phase chemistry) which enables us to prevent the released energy from going rampant and disperse in all available thermic degrees of freedom which are partly irrecoverable.
Expected features of radical batteries:
- recyclability: potentially excellent - depends on AP - system design
- durability: very high - like any AP product they slowly but unavoidably accumulate damage from natural high energy radiation - if one pushes the limits with power density spikes the battery components average lifetime will decrease exponentially
- measurability: excellent - one can count while zipping - there is zero self discharge
- power density: a lot higher than todays best motor-sport engines
- efficiency: very high - no thermal detour
- energy density: mediocre but sufficient - there's a lot of encapsulating structure per broken bond
- resource consumption: no scarce elements are needed
- health hazards: probably very low - no heavy metals are used
- danger (when crushed): probably flammable, unlikely explosive - the use of non burnable SiO2 & Al2O3 as structural material is preferable over diamond
Note that like in a hydrogen system a complete radical battery systems include both an energy storage and an energy converter (reactor). The generated driving motion can either be delivered to electrostatic motors / generators or in one or more steps merged to an axle of macroscopic scale. Its desirable to pack the functionally into different parts (e.g. different microcomponents). This allows adjustable storage to converter ratios.
Controlled molecular recombination
Radical batteries and entropic batteries do not allow for very high energy densities. To reach levels of gasoline one needs to turn to molecular energy storage substances. Since molecular energy storage substances often are in liquid gasseous or supercritical state entropy can effect efficiency and has to be considered. [Todo: take a closer look]
With cheap AP-production one can avoid the need for (dangerous) high energy densities. Street bound vehicles may simply get their power induced over an AP road infrastructure. What can't work without high energy density storages are planes and ships in water air and space (not considering sails).
Molecular hydrogen as energy medium
energy storage cells could be implemented as nano-capsules filled with hydrogen that is compressed to fluid like densities (around thousand atmospheres). Compression heat is an efficiency issue. Whether and how single molecules can be extracted into machine phase has to be analyzed. The lack of available bonds to hold the reactants might complicate matters. Single hydrogen atoms can be hold by two lone pairs of oxygen if the structure is charged H2O-H-OH2+ but oxygen atoms in machine phase structures (R2O-H-OR2) cant be charged that much.
Nanosystems 13.3.8. Mechanochemical power generation